In the recent decades, we have been witnessing rapid progress in integrated silicon photonic devices in a wide range of functionalities including modulators, photodetectors, optical switches and on-chip nonlinear processing.The tunability is fundamentallyessential to realize flexible photonic integrated circuits, which is typically realized by microheaters. However, to avoid the light-absorption loss induced by the metal, a thick silicon dioxide (SiO2) layer is typically introduced between the silicon waveguide and the metallic heater, which inevitably impedes the heat transportation and heat dissipation since the thermal conductivity of SiO2is low. Therefore, it remains a challenge to achieve both lower power consumption and faster response time. Several methods, such as free-standing waveguide structures, different doping level of the waveguide or employing graphene as heating material have been proposed in order to obtain both lower power consumption and faster response time.
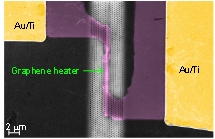
Figure 1. The schematic and SEM of the slow-light enhanced microheater.
Owing to many unique properties, such as a zero-band gap and tunable Fermi level, high carrier mobility and ultra-broad absorption bandwidth, graphene has been widely merged with nanophotonic structures to enhance the light-matter interaction.In addition to these broadly studied applications, the use of graphene as a heating material in close contact to the silicon waveguide can significantly improve the tuning efficiency due to graphene’s low optical absorption rate. Moreover, owing to the extremely high thermal conductivity of up to 5300 W•m-1•K-1, response times can be greatly reduced relative to devices with thick SiO2cladding between the metallic heater and the waveguides. More importantly, since the slow-light effect in the photonic crystal waveguide can effectively enhanced the light-matter interaction, Prof.JianjiDong from the Huazhong University of Science and Technology proposed that the combination of the graphene microheater and slow-light effect might be a feasible solution towards microheater’s low power consumption and fast tuning requirements.
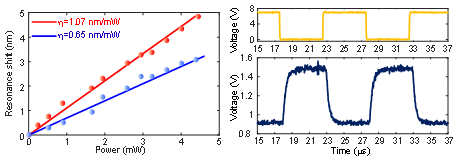
Figure 2.Performance of the slow-light enhanced graphene microheater.
Based on the theoretical analysis above, Prof. Dong cooperates with and Dr.YunhongDing and Prof. AsgerMortensonfrom DTU to fabricate the slow-light enhanced microheater. By incorporating a graphene microheater on a slow-light silicon photonic crystal waveguide (Fig. 1), we experimentally demonstrated an energy-efficient graphene microheater with a tuning efficiency of 1.07 nm/mW and power consumption per free spectral range of 3.99 mW. The rise and decay times (10% to 90%) were only 750 ns and 525 ns, which, to the best of our knowledge, are the fastest reported response times for microheaters in silicon photonics (Fig. 2). The corresponding record-low figure of merit of the device was 2.543 nW·s,which is one order of magnitude lower than results reported in previous studies.
Due to the widely required applications of microheaters in the photonic integrated circuits, the proposed concept of combining graphene microheater with photonic crystal waveguide provides a promising solution to reduce power consumption in many functional photonic devices, such as optical phase-array antenna, on-chip arbitrary waveform generators, optical switches, phase shifters, tunable filters and modulators.
On Feb. 9th2017,the work hasbeenpublished on by the Nature Communications (weblink:).This work is supported by the the Danish Council for Independent Research (DFF-1337−00152 and DFF-1335−00771) and the National Natural Science Foundation of China (Grant No. 61622502 and 61475052). The Center for Nanostructured Graphene is sponsored by the Danish National Research Foundation, Project DNRF103.