Laser powder bed fusion (LPBF) additive manufacturing (AM) is also known as selective laser melting (SLM), direct metal laser sintering (DMLS) or laser metal fusion (LMF), which offers a low-cost and time-efficient method to fabricate complex-shaped metal products and has been widely used in the aerospace, automotive and biomedical industries. LPBF is developing with the goal of fabricating parts with high performance and high efficiency. Laser power is the key factor to the efficiency, microstructure and performance in LPBF. However, there are limited reports regarding the laser-matter interaction in LPBF under high-power conditions.

Fig. 1. Time series snapshots of the evolution of the molten pool in LPBF as a function of the laser power. (a1-a5) No obvious large vapor depression and droplet column ejection occur for a laser power of 350 W. (b1-b5) A vapor depression forms in the molten pool after 50 μs, and a subthreshold ejection occurs where the column of droplet absorbs back into the molten pool with a laser power of 750 W. (c1-c5) A column of droplet oscillates and eventually breaks up into spatter particles at the rear of the molten pool with a laser power of 1150 W. (d1-d5) A large vapor depression and a column of droplet form before 50 μs, and the molten pool fluctuates violently with a laser power of 1550 W. The camera frame rate is 100 kfps with an exposure time of 1 μs. These snapshots are included in supplementary videos 1 with a selected FOV of 1.46 mm2.
In this work, based on the self-developed SLM equipment in Wuhan National Laboratory for Optoelectronics (WNLO), we developed a high-speed high-resolution imaging system to investigate the laser-matter interaction in LPBF. The highest spatial and temporal resolutions of this in situ characterization system are 2.5 μs and 4 μm/pixel, respectively. The core technology of this high-speed high-resolution imaging system has been authorized by the Chinese invention patent ZL 201710837757X. As shown in Fig. 1, based on high-speed high-resolution imaging observations, the molten pool characteristics and spatter behavior in LPBF of Inconel 718 with a high-power laser and a wide process window (from 350 W to 1550 W) are investigated, and the occurrence and evolution of the vapor depression and the protrusion in the molten pool are further discussed.
In LPBF, the molten pool occurs after the laser emission for several microseconds, followed by the generation of a metal vapor jet, the vapor depression, the protrusion and the droplet column ejection. The time of the first occurrence decreases dramatically (from ~102 μs to ~1 μs) with an increase in the laser input energy. As depicted in Fig. 2, the times of the first occurrence of the vapor depression tvd and the droplet column ejection tdc decrease with the increase in the laser power. tvd decreases from approximately 60 μs at a laser power of 750 W to 30 μs at a laser power of 1550 W, and tdc declines from approximately 140 μs at a laser power of 750 W to 40 μs at a laser power of 1550 W.
Furthermore, when the laser mode and spot size are kept constant, the laser power determines the time required for the abovementioned physical phenomena to occur, while the laser scan velocity determines whether the laser dwell time is sufficient for these phenomena to form. This study provides guidance for the optimization of the processing window of the melting regime in LPBF. This provides guidance for the optimization of the processing window of the melting regime (e.g. conduction mode or keyhole mode) in LPBF.

Fig. 2. The calculated critical laser dwell times for local melting tmelting and boiling tboiling. The times of the first occurrence of the vapor depression tvd and the droplet column ejection tdc derived from the high-speed high-resolution observations. The laser beam dwell time td of 224.9 μs, 149.9 μs and 112.4 μs at velocities of 1000 mm/s, 1500 mm/s and 2000 mm/s, respectively, are represented with black dashed lines. (A base 2 logarithmic scale is used for the plot. tvd and tdc are absent at laser power of 350 W, since no obvious large vapor depression and droplet column ejection occur).
During LPBF, spatter is the material ejected from the molten pool and its vicinity. The spatter can be categorized as a hot droplet spatter from the unstable molten pool due to the recoil pressure and Marangoni effect, and a cold powder spatter because of the vapor-induced entrainment from the denudation zone adjacent to the molten track. The large particles land back in the powder bed and have the potential to alter the local particle distribution with an increased possibility of producing porosity. Therefore, the spatter creates a secondary powder system, which is generally considered to be a detrimental process in LPBF. To further study the effects of laser power on the spatter behavior, more than 100 spatter ejection trajectories were traced for laser powers from 350 W to 1550 W; the average ejection velocity and average ejection angle for each spatter trajectory are statistically demonstrated in Fig. 3. The majority of spatters eject out backward, while a few spatters have a horizontal velocity component along the laser direction. The average ejection velocity and average ejection angle increase with the laser power. Large spatters are also likely to be generated with a high-power laser. The dominant mechanism of large spatter generation can be interpreted as being due to the instability of the molten pool driven by vapor recoil pressure and Marangoni effect, hot spatter collisions during flight and powder particles agglomerated on the substrate or solidified region before being ejecting out driven by the metal vapor entrainment.
This work provides a deep understanding of the laser-matter interaction under high-power conditions, which is of vital significance to the physical basis during the high-power LPBF and the properties of a part that is processed by LPBF. This work lays a foundation for the construction and development of the platform of "Extreme Laser-Material Interaction" in Wuhan National Laboratory for Optoelectronics.
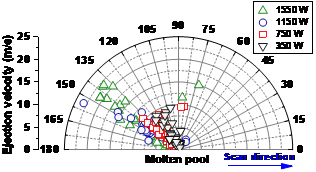
Fig. 3. Polar plot of the average ejection velocity and average ejection angle of the spatters for different laser powers. Each point on the polar plot represents a spatter ejection trajectory. More than 100 spatter ejection trajectories are traced from t=700 μs to t=2100 μs with the full FOV of 3.66 mm2. The laser beam moves from left to right, and the molten pool is shown at the origin of the polar plot, with an ejection average angle of q=0° for the direction of the laser movement and 90° for the direction of the deposition.
On July 3rd, this work “High-power laser-matter interaction during laser powder bed fusion” has been published on the international journal Additive Manufacturing (2018 IF: 7.173, 1st place in Engineering, Manufacturing of JCR). Dr. Jie Yin is the first author of this paper. Prof. Haizhong Zhu and Prof. Xiaoyan Zeng are the corresponding authors. M.D. student Liangliang Yang and Ph.D. student Xu Yang participant this work. Prof. Zemin Wang and Assoc. Prof. Dengzhi Wang participate in the guidance.
The authors gratefully appreciate the financial supports from National Key Technologies R&D Program of China (2017YFB1103800), National Natural Science Foundation of China (61805095 and 61475056) and China Postdoctoral Science Foundation (2018M642832). We also acknowledge the support and recommendation of the conference committee of the First International Conference on Research Advances in Additive Manufacturing (RAAM).
Reference: J. Yin, L. Yang, X. Yang, H. Zhu, D. Wang, L. Ke, Z. Wang, G. Wang, X. Zeng, High-power laser-matter interaction during laser powder bed fusion, Additive Manufacturing 29 (2019) 100778.
Full text link: https://doi.org/10.1016/j.addma.2019.100778