Anode-free batteries with initial-Li-free anode could avoid the direct use of metallic Li and maximize the battery energy density, where the cathodes provide active Li, and metallic Li is deposited on current collectors during the battery charge process and dissolved during the subsequent battery discharge process. However, they encounter fast failure due to the inferior electrochemical reversibility. One main reason is the fast inactive Li accumulation caused by the coupling of side reactions and mechanics during complicated Li plating/stripping processes, among which, mechanical stability of plated Li on current collector is crucial but often ignored. Thus, it is urgent to suppress the formation of inactive metallic Li, and improve mechanical stability and electrochemical reversibility of initial-Li-free anode.
Yongming Sun’ group proposed a stable lithium-metal alloy interphase for Li plating to sharp the Li deposition morphology and enhancedthe connection with deposited Li and achieved good electro-mechanical stability. The Li-metal alloy possessed reduced Li nucleation energy barrier and strong bonding with metallic Li, which enabled dense and chunky Li deposits with firm connection with the substrate in sharp contrast the fully peeling off of metallic Li for bare Cu after 50 Li plating/stripping cycles. A Li-Sn alloy-modified Cu anode displayed stable Li plating/stripping cycling with average Coulombic efficiency of 94.1% for 400 cycles at 1 mA cm–2and 1 mAh cm–2, significantly outperforming the bare Cu electrode. As a demonstration, in an anode-free cell with Li rich Mn-based cathode, 16.7% increase in capacity and 14.1% increase in capacity retention for 20 cycles were achieved using alloy-modified anode. This sheds new insights on the improvement of dynamic mechanical stability between Li deposits and current collector by regulating Li plating and its connection with the current collector and guides the design of high-performance initial-Li-free anode.
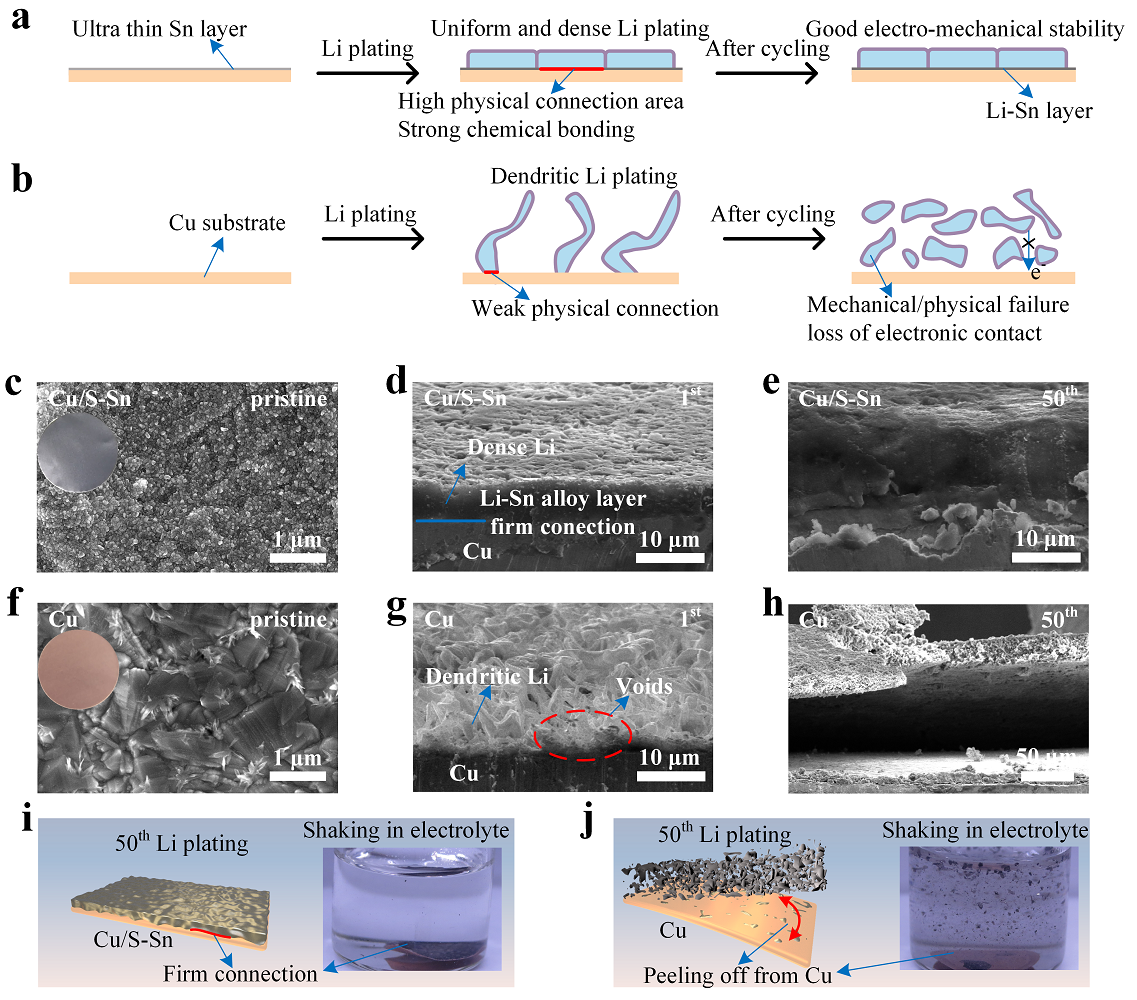
Fig. 1.Schematic diagram of the initial Li plating process and the evolutions of the morphology and structure of the (a) Cu/S-Sn and (b) Cu electrodes on cycling. Top-view SEM images of the (c) pristine Cu/S-Sn and (f) Cu electrodes. The inserts were the corresponding digital photos. Cross-section SEM images of the (d) Cu/S-Sn and (g) Cu electrodes after the 1stLi plating. Cross-section SEM images of the (e) Cu/S-Sn and (h) Cu electrodes after the 50thLi plating. Schematic diagrams of the evolution of (i, left) Cu/S-Sn and (j, left) Cu electrodes on cycling. The digital photos of the (i, right) Cu/S-Sn and (j, right) bare Cu electrodes at the 50thLi plating after shaking in electrolyte for 5 seconds. The test was conducted after Li plating at 1 mA cm–2for 1 hour followed by charging to 1 V at same current density to make Li strip for each cycle.
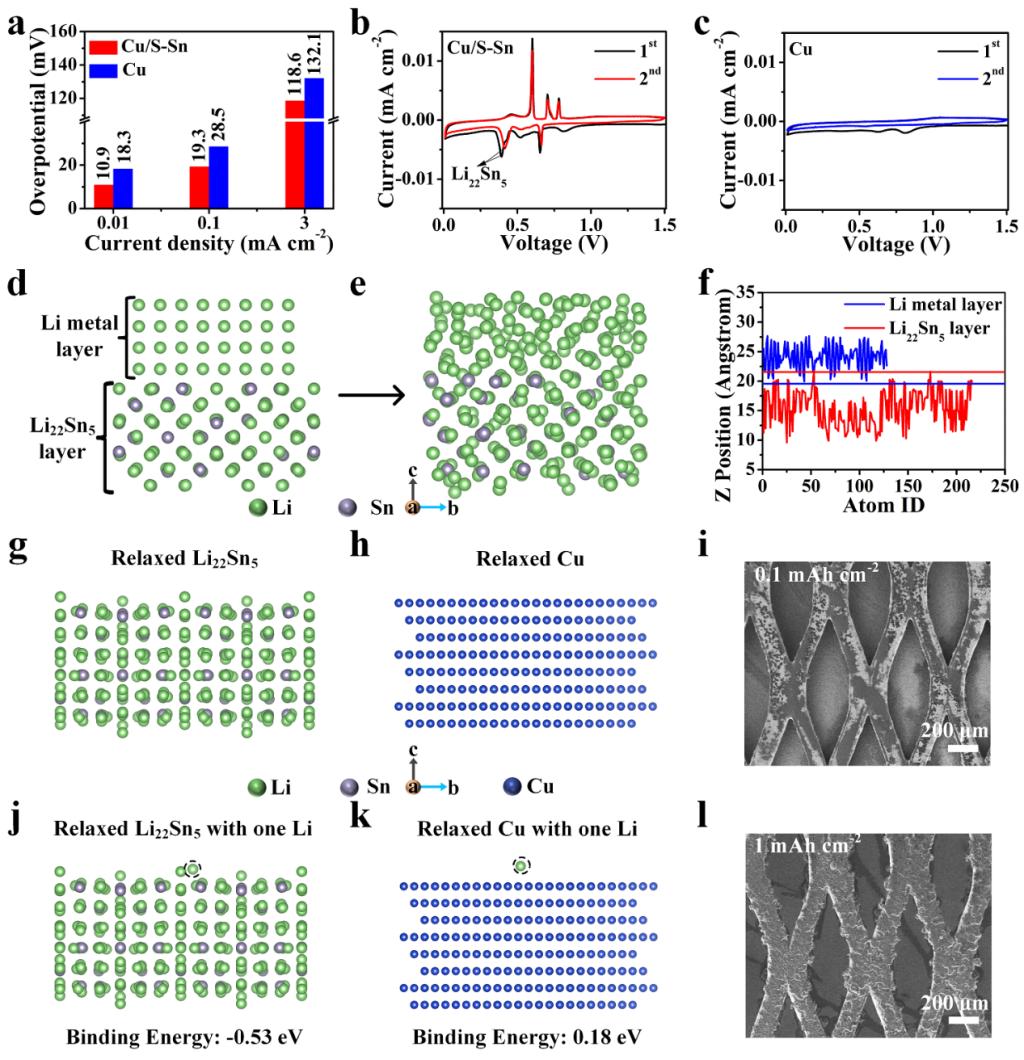
Fig. 2.(a) Comparison of the Li nucleation overpotential on Cu/S-Sn and Cu electrodes at different current densities. (b, c) CV results of the Li||Cu/S-Sn and Li||Cu cells, respectively. (h-j) Simulation results of the interface diffusion between Li metal layer and Li22Sn5layer. (d-f) Simulation results of the interface diffusion between Li metal layer and Li22Sn5layer. (g, j) Calculation results of binding energy between Li and Li22Sn5. (h, k) Calculation results of binding energy between Li and Cu. (i) SEM images of electrodes of Cu mesh with S-Sn on Cu foil after plating of 0.1 and (l) 1 mAh cm–2Li at 0.1 mA cm–2.
Related work has been published on Energy Storage Materials, with the title of Li plating on alloy with superior electro-mechanical stability for high energy density anode-free batteries. The first unit for this research is Wuhan National Laboratory for Optoelectronics,Huazhong University of Science and Technology, and it has been funded by National Natural Science Foundation of China.
See the paper at Energy Storage Mater., 2022, https://doi.org/10.1016/j.ensm.2022.04.009; Volume 49, August 2022, Pages 135-143.