Metallic Lithium (Li) is regarded as one of the most promising anode materials for next-generation high energy density battery technologies since it delivers the highest theoretical specific capacity (3860 mA h g−1) and lowest reduction potential (−3.04 V vs. standard hydrogen electrode) among the existing Li-based chemistries. The uneven electrochemical reactions of Li metal anode is one of the main reasons that hinder its application in rechargeable high energy density batteries. Great progress has been achieved in homogenizing electrochemical reactions of Li metal anode based on laboratory-scale coin cells or single-layer pouch cells, whose experimental parameters and working environments are far from practical batteries, and thus cannot provide direct guidance for their improvement. In fact, practical batteries (eg., Ah-level laminated pouch cells) usually delivered much faster electrochemical failure of Li metal electrode in comparison to coin cells. Therefore, intensive efforts should be devoted to understanding and addressing the uneven electrochemical reactions over large-scale electrodes in practical Li metal batteries and extending their cycle life.
Yongming Sun’s group analyzed the difference in electrochemical Li plating/stripping behavior between coin cell and Ah-level laminated pouch cell, and revealed the intrinsic inhomogeneity of electrochemical reactions caused by pouch cell structure, which explained the much faster electrochemical failure of Li metal electrode in laminated pouch cell in comparison to coin cell. Using Li/Li-Sn composite electrode with an ionically conductive framework to replace pristine Li electrode, it has been demonstrated the significant role of Li alloy composite anode design in eliminating the negative effect of inhomogeneous electrochemical reactions that different local electric field/current cause. Porous and dendritic Li deposits with loose structure was observed in pristine Li metal electrode with a size of 8 × 11 cm after cycling, where the near-tap and the central regions with close connection with the current collector showed more severe corrosion than the edge region due to the locally amplified current density, although cracks still spread over the entire edge region. In sharp contrast, uniform Li deposits with a dense structure were observed over different regions of a Li/Li–Sn alloy composite anode, as well as suppressed parasitic reactions and gas evolution, supporting the positive effect of Li alloy composite anode design in homogenizing electrochemical reactions, and prolonging the lifespan in Ah-level laminated pouch cell configuration.
As a demonstration, a 1.2 Ah SPAN||Li/Li–Sn pouch cell exhibited steady charge/discharge performance over 100 cycles with a capacity retention of 87% at 600 mA (1 C = 1200 mA, a cathode areal capacity of 3.4 mA h cm−2), far outperforming the 1.2 Ah SPAN||Li pouch cell that lost all its capacity after 40 cycles. This work discloses the uneven electrochemical reactions that pouch cell structure causes and provides basic insights for understanding the uneven Li plating/stripping behavior and deteriorated electrochemical performance caused by the difference in local electrochemical environment in practical Li metal pouch cells.
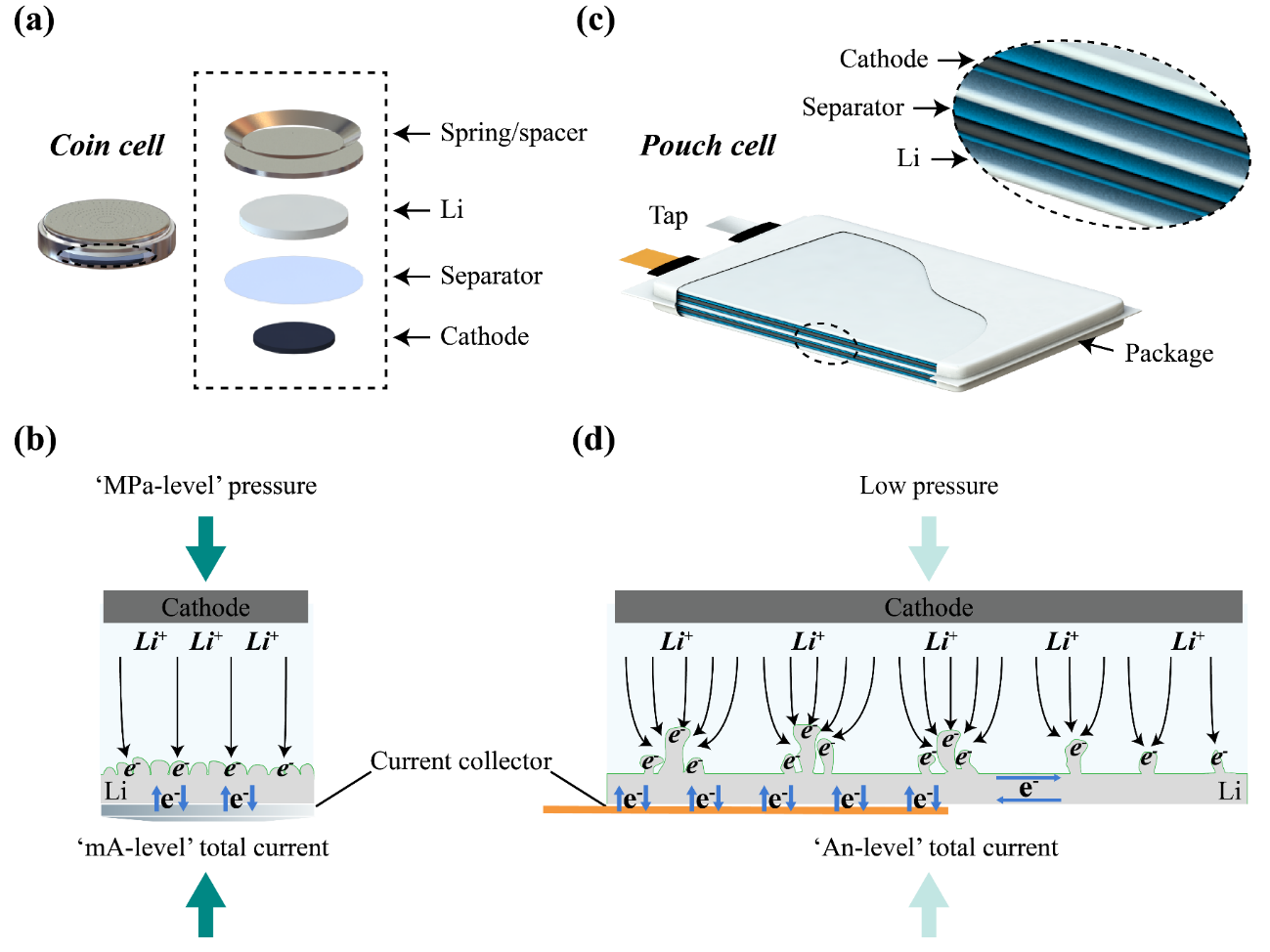
Figure 1. Comparison of coin cell and pouch cell configurations. (a and b) Schematic illustration of coin cell and Li anode with mA-level total current, MPa-level pressure, and a spring/spacer component that covers the entire metallic Li electrode and severs as a current collector and pressurizer, enabling relatively uniform electrochemical reactions. (c and d) Schematic illustration of pouch cell and Li anode with An-level total current and low pressure, where Cu foil current collector partially connects with the metallic Li anode (the near-tap and the central regions), leading to uneven electrochemical reactions.
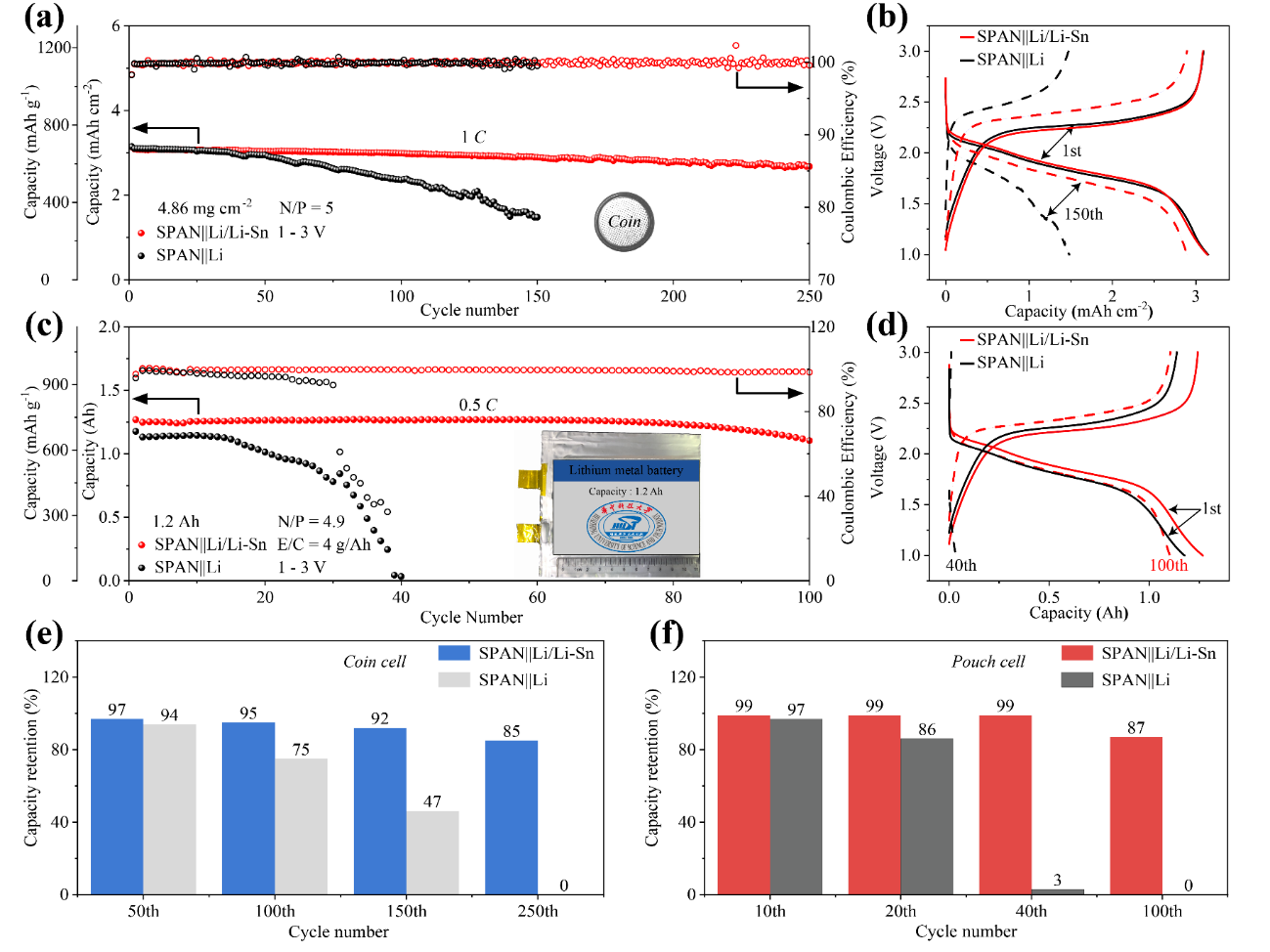
Figure 2. (a) Electrochemical performance of SPAN||Li and SPAN||Li/Li-Sn coin cells at 1 C. The inset is a photograph of the as-prepared coin cell. (b) Voltage profiles of SPAN||Li and SPAN||Li/Li-Sn coin cells for selected cycles in Fig. 2a. (c) Electrochemical performance of 1.2 Ah SPAN||Li and SPAN||Li/Li-Sn laminated pouch cells at 600 mA. The inset is a photograph of the as-prepared 1.2 Ah pouch cell. (d) Voltage profiles of 1.2 Ah SPAN||Li and SPAN||Li/Li-Sn laminated pouch cells for selected cycles in Fig. 2c. (e) Comparison of capacity retention for SPAN||Li and SPAN||Li/Li-Sn coin cells for selected cycles in Fig. 2a. (f) Comparison of capacity retention for 1.2 Ah SPAN||Li and SPAN||Li/Li-Sn laminated pouch cells for selected cycles in Fig. 2c.
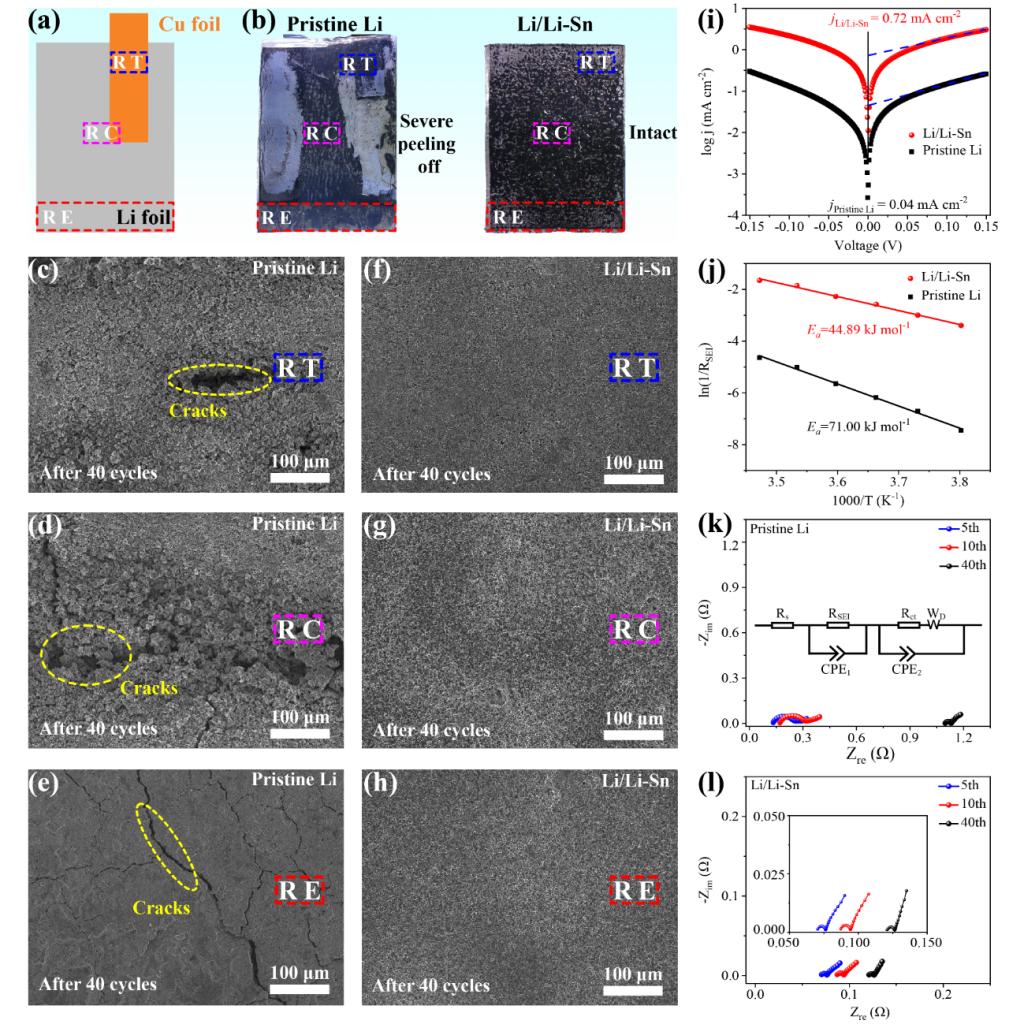
Figure 3. (a) Schematic illustration of the anode construction where three selected regions were labeled with indicating boxes, including the near-tap (RT), the central (RC), and the edge (RE) regions. (b) Digital photos of pristine Li (left) and Li/Li-Sn alloy composite (right) electrodes from cycled laminated pouch cells (1.2 Ah, 600 mA after 40 cycles). The corresponding top-view SEM images of (c-e) pristine Li and (f-h) Li/Li-Sn alloy composite electrodes in (c and f) RT, (d and g) RC, and (e and h) RE regions. (i) Tafel curves of pristine Li and Li/Li-Sn symmetric cells. (j) Apparent activation energy of Li+ transfer through SEI layer for pristine Li and Li/Li-Sn alloy composite electrodes. Nyquist plots of (k) pristine Li and (l) Li/Li-Sn alloy composite electrodes after 5, 10, and 40 cycles.
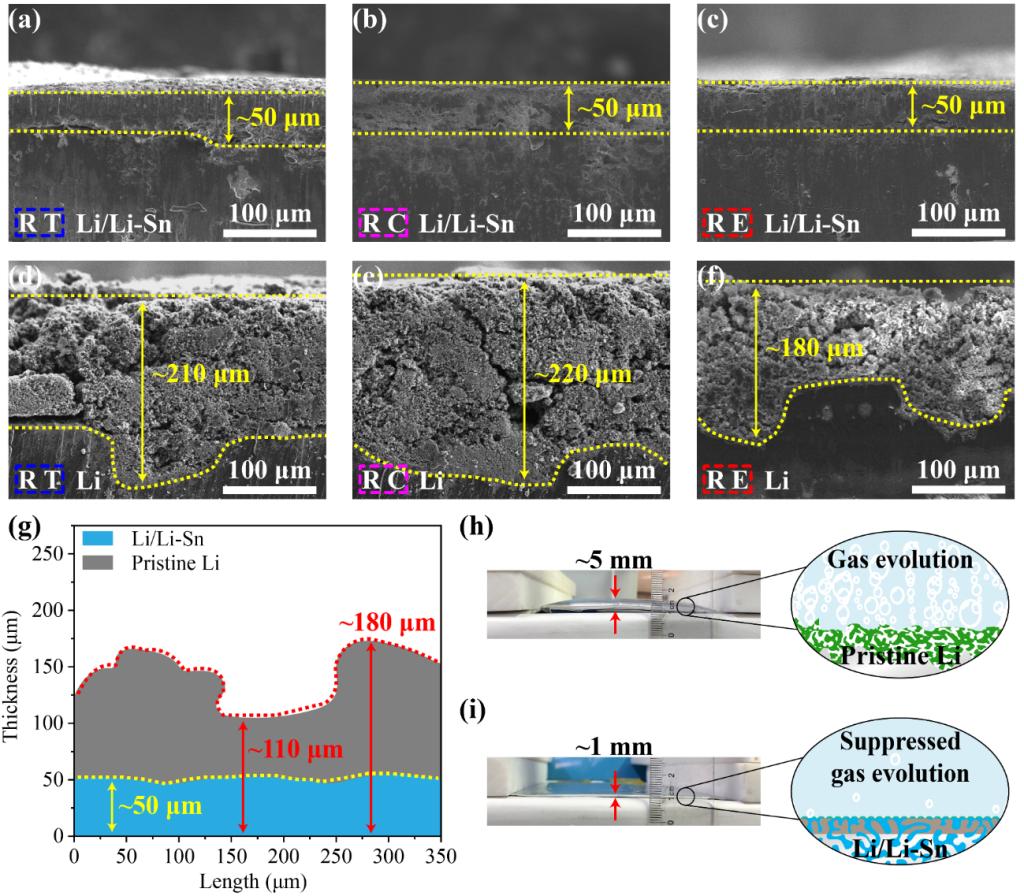
Figure 4. Cross-sectional SEM images of (a-c) Li/Li-Sn alloy composite and (d-f) pristine Li electrodes, obtained from the disassembled 1.2 Ah laminated pouch cells after 40 cycles in (a, d) RT, (b, e) RC, and (c, f) RE regions. (g) Thickness fluctuation of reaction layers for Li/Li-Sn alloy composite and pristine Li electrodes in RE region. Optical images and corresponding schematic illustrations of gas evolution of (h) SPAN||Li and (i) SPAN||Li/Li-Sn laminated pouch cells after 40 cycles.
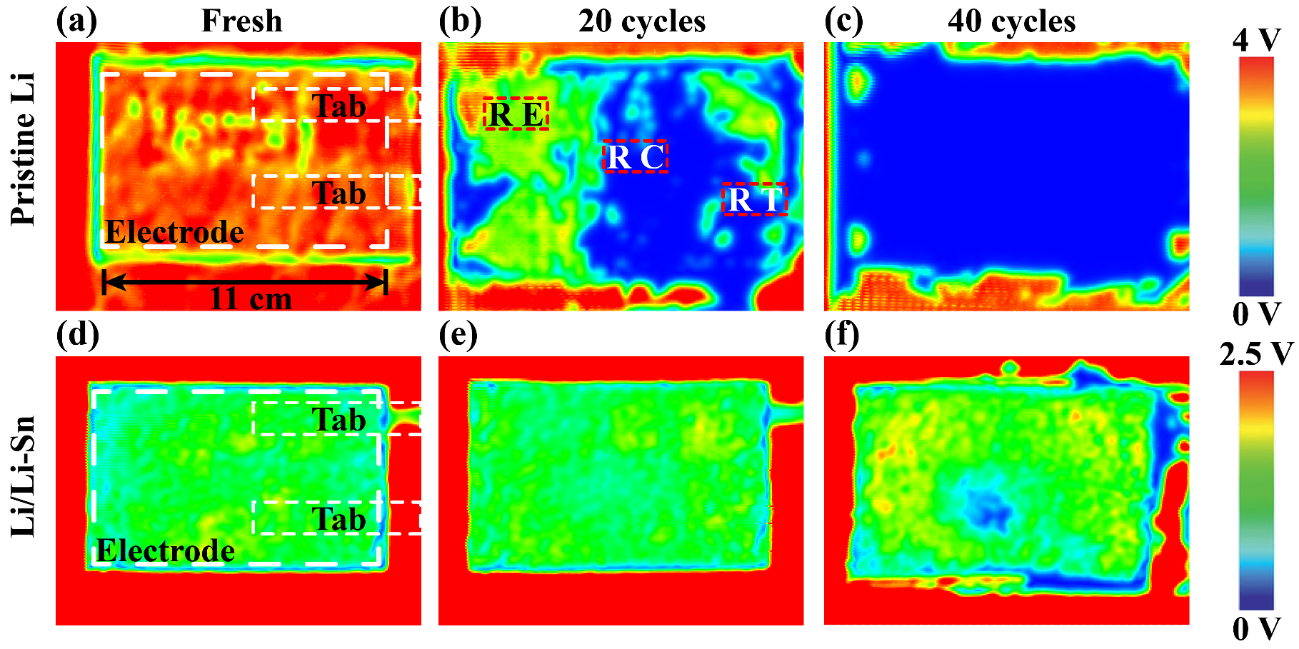
Figure 5. Ultrasonic transmission mappings of (a-c) SPAN||Li and (d-f) SPAN||Li/Li-Sn laminated pouch cells with large electrode size of 8 x 11 cm (a and d) before cycling, (b and e) after 20 cycles, and (c and f) 40 cycles at 0.5 C.
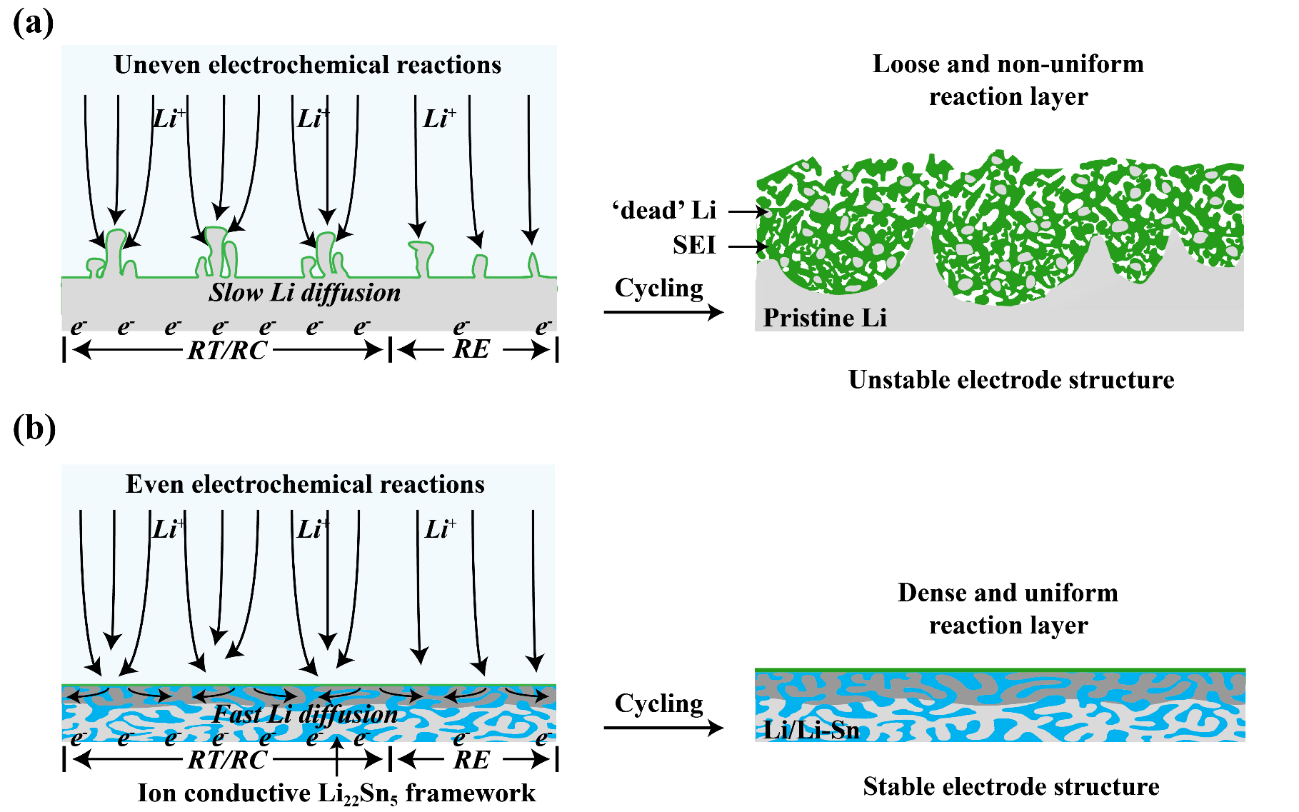
Figure 6. Schematic illustration of the proposed evolution of (a) pristine Li and (b) Li/Li-Sn alloy composite electrodes in laminated pouch cells during cycling. The enhanced ion transport property and robust Li-Sn alloy framework structure of Li/Li-Sn alloy composite electrode enable fast lithium diffusion and stable electrode structure, leading to extended cycle life.
Related work has been published on Advanced Functional Materials (https://doi.org/10.1002/adfm.202210669) on November 27, 2022, with the title Revealing the intrinsic uneven electrochemical reactions of Li metal anode in Ah-level laminated pouch cells. The first unit for this research is the Wuhan National Laboratory for Optoelectronics, Huazhong University of Science and Technology, and it has been funded by the National Natural Science Foundation of China.