The high capacity (3860 mAh g-1) and low working potential (-3.04 V versus standard hydrogen electrode) of metallic Li make it a promising anode material for next-generation high-energy rechargeable batteries. However, its implementation is impeded by its limited lifespan. Among the factors that degrade the electrochemical performance of Li metal anodes, Li/electrolyte side reactions are the most dominant. The creation of a fluorinated interphase has emerged as an effective strategy for relieving the side reactions and achieving reliable Li metal batteries.
Recently, Professor Yongming Sun’s group at Wuhan National Laboratory for Optoelectronics, Huazhong University of Science and Technology, propose the creation of a LiF-rich SEI through a novel dissolution-reprecipitation mechanism of LiF for Li metal protection, which can avoid the occurrence of undesirable side reactions caused by fluorinated solvents or fluorine-donating anions and is conceptually different from the electrochemical/chemical decomposition of electrolyte components (Figure 1a). For validation, a Li/Li22Sn5/LiF ternary composite was investigated, where the as-formed LiF-rich SEI locked the active Li metal from a corrosive electrolyte. As expected, the Li/Li22Sn5/LiF composite delivers an impressive average Coulombic efficiency (~99.2%) upon cycling in a carbonate electrolyte compared to ~96% for a regular Li metal anode at 1 mA cm-2 and 1 mAh cm-2 and a long cycling life of over 1600 h at 1 mA cm-2 and 2 mAh cm-2. Paired with a LiCoO2 cathode with an areal capacity of 4.0 mAh cm-2, the full cell demonstrates a remarkable capacity retention of ~91.1% for 100 cycles at 0.5C with a low negative/positive ratio (N/P ratio) of 2:1. This design is conceptually different from the design employing the widely used fluorine-containing electrolyte additive to introduce fluorine species in the SEI and provides an alternative approach to realize reliable Li metal batteries.
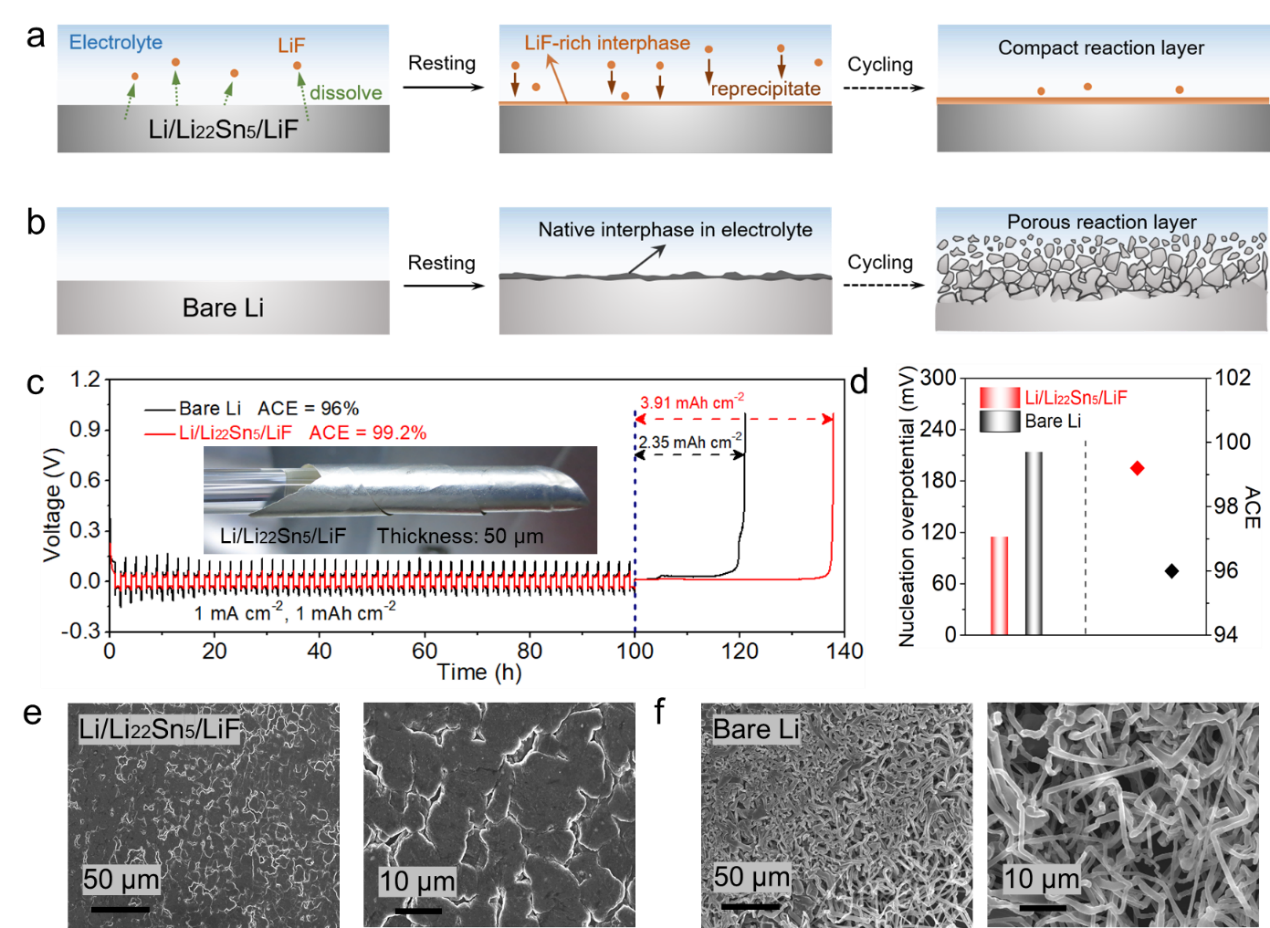
Figure1. (a) Schematic of LiF dissolution-reprecipitation mechanism-enabled interphase protection for Li/Li22Sn5/LiF electrode on cycling. (b) Schematic forthe evolution of bare Lielectrode on cycling. (c) Li plating/stripping voltage profile of Li/Li22Sn5/LiF and bare Li electrodes at 1 mA cm−2 and 1 mAh cm−2 for 50 cycles and subsequent Li stripping at 0.2 mA cm-2. The inset shows a digital image of 50 µm-thick Li/Li22Sn5/LiF foil. (d) Comparison of nucleation overpotential and ACE of Li/Li22Sn5/LiF and bare Li electrodes. Top-view SEM images of (e) Li/Li22Sn5/LiF and (f) bare Li electrodes after electrochemical plating of metallic Li at 1 mA cm−2and 1 mAh cm−2.
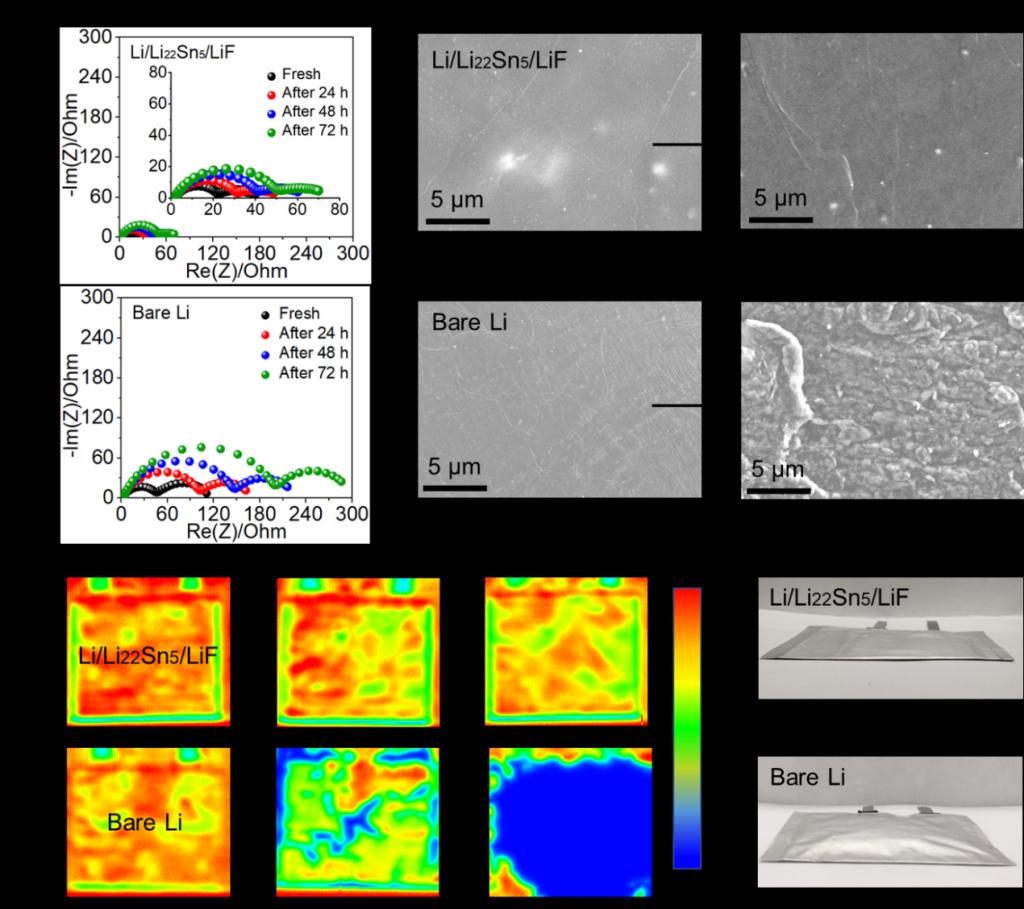
Figure 2. Nyquist plots of (a) Li/Li22Sn5/LiF and (b) bare Li symmetric cells after resting at 60 °C for different times. SEM images of (c) fresh Li/Li22Sn5/LiF and (d) bare Li samples before and after resting in electrolyte at 60 °C for 14 d. Ultrasonic transmission mappings of (e) Li/Li22Sn5/LiF and (f) bare Li symmetric pouch cells during resting at 60 °C. Optical photograph of (g) Li/Li22Sn5/LiF and (h) bare Li symmetric pouch cells after resting at 60 °C for 14 d. The size of electrodes used in pouch cells is 4 × 5 cm. Gas evolution is observed for the bare Li symmetric pouch cells.
This work is reported on Advanced Materials titled Locking active Li metal through localized redistribution of fluoride enabling stable Li metal batteries (Advanced Materials, 2022, 202207310. https://onlinelibrary.wiley.com/doi/10.1002/adma.202207310). Guocheng Li and Xiangrui Duan at Wuhan National Laboratory for Optoelectronics contributed equally to this work. Prof. Yongming Sun lead this work. Prof. Zhao Cai and Yue Shen at Huazhong University of Science and Technologyalso contributed to this work.